Exploring the Theoretical Foundations of Quantum Computing
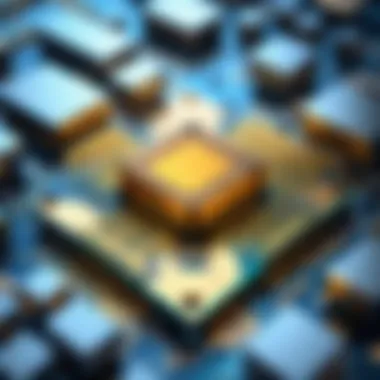
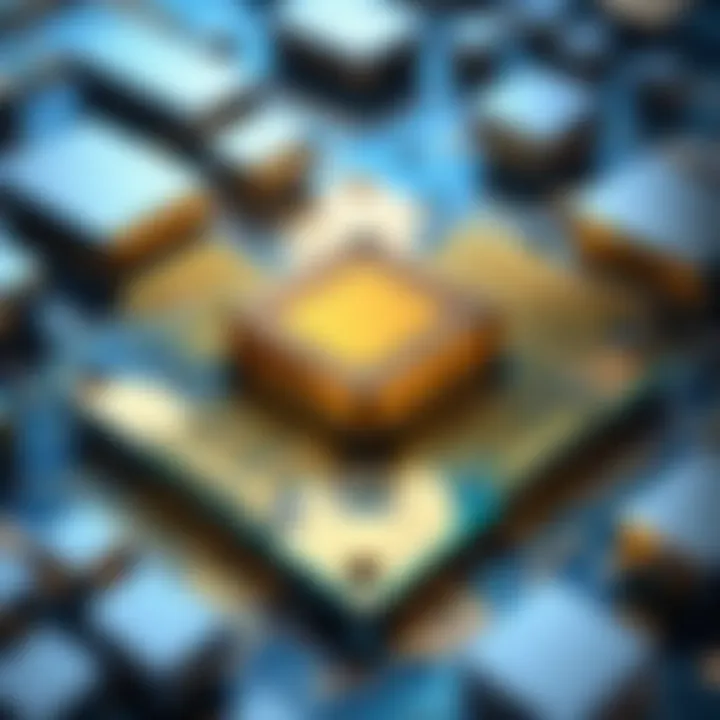
Intro
Quantum computing is a topic that continues to attract significant interest from both the academic world and the tech industry. Its theoretical foundations challenge the way we interpret computation itself. Unlike classical computing, where information is processed in bits that are either 0 or 1, quantum computing uses qubits that hold much greater information potential. This leads to capabilities that could revolutionize fields ranging from cryptography to drug discovery.
It is essential to grasp the fundamental principles that govern this technology. Concepts such as superposition, entanglement, and quantum interference will be examined in detail. Also, understanding how these principles differentiate quantum computing from traditional computing is crucial to appreciating its innovative abilities.
Tech Trend Analysis
Overview of the current trend
The rise of quantum computing is one of the most mentioned topics in technological discourse today. Major corporations like IBM, Google, and Microsoft are pouring resources into quantum research and development. Governments worldwide are not lagging behind; they are investing heavily in quantum initiatives. The trend indicates a global race toward quantum supremacy, showcasing the technology's potential applications in diverse sectors.
Implications for consumers
For consumers, the implications of this development are just beginning to surface. Quantum computing may lead to faster processing speeds and enhanced features in personal computing devices. Privacy and security could also see substantial improvements, as the way data is encrypted could be radically transformed. New advancements in secure communication may very well originate from this technology.
Future predictions and possibilities
Looking ahead, quantum computing has the potential to solve problems intractable for classical computers. Fields such as optimization, materials science, and artificial intelligence stand to benefit significantly. Industry professionals believe that within the next decade, some commercial applications may become viable. Thus, staying informed about trends in quantum advancements is vital for anyone in the tech field.
“The real world applications of quantum computing may leave a monumental impact on the very acceleration of technology.” - Expert in Quantum Theory
Key Concepts in Quantum Computing
To fully understand quantum computing, several concepts need deeper exploration.
- Quantum Bits (Qubits): The building blocks of quantum information.
- Superposition: Enables qubits to represent both 0 and 1 simultaneously.
- Entanglement: A unique correlation between qubits that leads to new approaches in computing efficiency.
In summary, knowledge of these fundamental principles reveals the transformative potential lurking in quantum computing.
Careful observation will be necessary as future research unfolds and the adaptation of this technology begins. The unpredictability of breakthroughs in quantum mechanics could affect both technical paradigms and everyday life.
Prolusion to Quantum Computing
Quantum computing stands at the crossroads of technology, mathematics, and physics. This article focuses on the multifaceted landscape of quantum computing, illuminating its theoretical foundations and searching into its capabilities.
What is Quantum Computing?
Quantum computing represents a paradigm shift in computation. Unlike classical computing, which uses bits as the smallest unit of information, quantum computing utilizes quantum bits or qubits. The distinction is critical; while conventional bits can only be in one state, either 0 or 1, qubits can exist in multiple states simultaneously. This principle allows quantum computers to process a vast amount of information at unprecedented speeds.
In essence, quantum computing encapsulates complex phenomena governed by quantum mechanics. It involves concepts like superposition and entanglement, which do not have direct analogs in classical computing. As this technology matures, it has the potential to revolutionize various sectors from cryptography to artificial intelligence, offering solutions that were previously unattainable due to the limitations of traditional systems.
Importance of Quantum Computing
The significance of quantum computing extends far beyond computational speed. Its importance is rooted in several aspects:
- Enhanced Processing Power: Quantum computers can solve problems that are infeasible for classical computers owing to their immense processing capabilities.
- Novel Applications: Fields such as materials science, cryptography, and complex system modeling can benefit enormously from quantum approaches, paving the way for innovations that could redefine industries.
- Competitive Edge: As nations and corporations invest heavily in quantum research, understanding its mechanisms and potential applications is vital for anyone engaged in technology sectors.
- Academic and Practical Research: The ongoing research around quantum algorithms and architectures not only enriches academic perspectives but also has practical relevance for industries striving to harness advanced computational methods.
- Climate modeling and drug discovery: These areas stand out; they require simulations and calculations beyond the reach of current classical computers.
In summary, the journey into quantum computing invites both curiosity and strategic thinking, suggesting it will have broader implications for society. The aim is not merely to grasp a new field but to understand its impact on our future.
The drive towards quantum computing harbors potential that may exceed our current comprehension — a chance to reimagine the very fabric of technology as we know it.
Fundamental Principles of Quantum Mechanics
The study of quantum mechanics is essential to understanding quantum computing. These principles govern the behavior of particles at atomic and subatomic levels, shaping the very foundation of quantum information processing. Recognizing these fundamental elements sheds light on the mechanisms driving quantum computing technologies and provides insight into their capabilities and limitations.
Quantum Bits (Qubits)
Quantum bits, or qubits, represent the fundamental unit of quantum information. Unlike classical bits, which can exist only in one of two states: 0 or 1, qubits can be in a state of positivity or negativity. This capacity oen access to a spectrum of possibilities due to their unique nature.
- Superposition: One of the most innovative qualities of qubits is their ability to exist in multiple states at once. This allows quantum computers to process vast amounts of information concurrently, greatly exceeding classical counterparts.
- Manipulation: Qubits can be manipulated using quantum gates, which transfer quantum states and perform vast calculations at speeds previously unrealizable. Moreover, quantum circuits are architected to enable complex operations while maintaining coherence and minimizing decoherence effects.
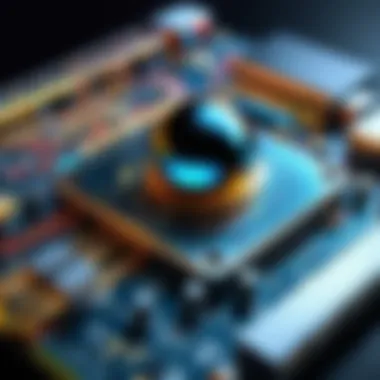
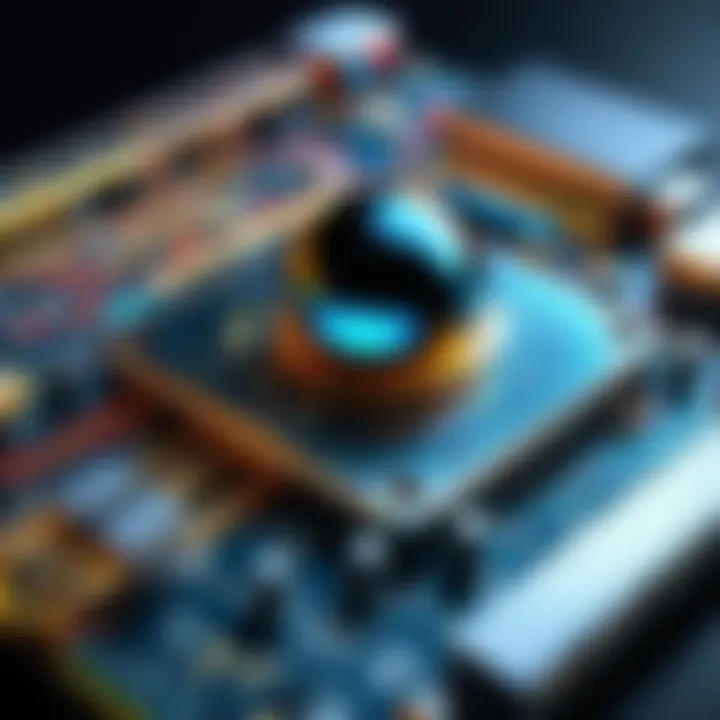
Overall, qubits illustrate the basic tenets of quantum computing, serving a key role in its potential applications.
Superposition and its Significance
Superposition is a critical tenet of quantum mechanics that features the principle of qubits ability to assume multiple states simultaneously. This phenomenon allows a quantum computer to perform calculations multiple times in parallel rather than sequentially, as classical systems typically do.
- This can represent multiple binary configurations, enabling faster data processing.
- The parallelism inherent in superposition positions quantum computers to tackle tasks that would typically take considerable troubleshooting and manipulations unprecedented by classical computing systems.
As a result, superposition elevates quantum computing's potential to unravel complex problems in optimization, cryptography, and artificial intelligence applications beyond thereach of classical devices.
Entanglement Explained
Entanglement is another core principle of quantum mechanics wherein pairs of qubits become interrelated, maintaining an inherent correlation regardless of relative distance. This means that the state of one qubit instantaneously affects the other, even when they are separated by vast distances.
- Unique Computation Capability: This property enables quantum computers to solve problems involving massive data points efficiently. The interconnectedness of entangled qubits minimizes reliance on extensive material systems, streamlining computations.
- Quantum Teleportation: Entanglement forms the basis for quantum teleportation—allowing instantaneous transmission of quantum information between distant points—showcasing capabilities1 that fundamentally differ from classical systems.
Entanglement unlocks paths toward enhanced computational power, enabling algorithms reflective of efficient symbiosis across data strands. Its relevance extends across diverse fields of research, from cryptography advancements to the artificial bolstering of AI systems.
"Understanding the core principles of quantum mechanics is essential for appreciating the potential of quantum computing. These principles unveil a realm of opportunity and innovation that redefines computation practices."
Differences Between Classical and Quantum Computing
In understanding the advances in computing technology, one must examine the critical contrasts between classical and quantum computing. The significance of this discourse lies in grasping the inherent characteristics that define each model. This section presents clear examples of how quantum computing diverges from classical approaches, illustrating not just different technologies, but different paradigms for processing information as well.
Processing Information
Classical computing relies on binary bits, fundamental units tasked with either a value of zero or one. These bits form the foundation for data processing. When processing information, classical computers operate linearly; they follow precise sequences of instructions to arrive at the desired output. This system can work effectively for many calculations and data handling tasks.
In contrast, quantum computing uses quantum bits, or qubits. A specific trait of qubits is their ability to exist in states other than zero and one simultaneously. This principle, known as superposition, allows quantum computers to handle a vast amount of operations concurrently. Consequently, instead of having one processing pathway, quantum systems can evaluate multiple possibilities at once, potentially solving complex problems significantly quicker than their classical counterparts.
Key Differences in Processing:
- Information Representation: Classical computers work with bits, whereas quantum computers use qubits.
- Operational Speed: Quantum processors can perform multiple calculations at the same time due to the nature of superposition, making them faster in dealing with specific complex problems.
- Complex Problem Solving: Tasks involving optimization problems can be more effectively addressed with quantum methods due to simultaneous evaluations of potential solutions.
Problem Solving Capabilities
The problem-solving capabilities of each computing type reveal further key differences. Classical computers excel at traditional algorithms and can digest significant amounts of data using methodologies like those in structured query languages. However, efficiency can diminish when solving problems defined by combinatorial requirements or tasks involving extensive searching of unsorted datasets.
On the other hand, quantum computing paves new paths with algorithms specifically designed for the unique attributes of quantum mechanics. A highlighted example is Shor's algorithm which enables the factorization of large integers more efficiently than any known classical algorithm. This innovation presents profound meaning for areas such as cryptography, confronting longstanding encryption methods founded on classical limitations.
For practical illustration:
- Additive tasks: Classical computers can handle these effectively.
- Search operations: Classical processes can struggle where the dataset grows dramatically, whereas Grover's algorithm allows quantum systems to search through extensive databases with fewer steps.
Quantum computers redefine how problem-solving approaches events, optimizing solutions through their distinct processing capabilities.
Quantum Algorithms
Quantum algorithms sit at the heart of quantum computing, providing crucial frameworks that leverage the unique properties of quantum mechanics to solve complex problems. The evolution of these algorithms marks a significant departure from classical algorithms, drawing on the principles of superposition and entanglement inherent to qubits. As quantum computers mature, the importance of these algorithms grows, driving real-world applications that traditional computing cannot efficiently address. They transition the theoretical concepts of quantum physics into practical tools leading to advancements in diverse fields like cryptography and optimization problems.
Intro to Quantum Algorithms
Quantum algorithms are designed specifically for quantum computing. Unlike classical algorithms, which rely on binary bits, quantum algorithms take advantage of quantum bits or qubits, which can exist in multiple states simultaneously. This property enables them to process vast amounts of information concurrently. One key feature is their efficiency. Quantum algorithms, through their unique operations, can solve problems in polynomial time that would typically take classical algorithms an infeasible amount of time.
Some notable quantum algorithms include Shor's Algorithm and Grover's Algorithm. These algorithms provide significant benefits in specific domains, like factorization and database searching. Their development signals a shift in computational capabilities and raises essential considerations about the future of technology and security. Quantum algorithms will continue to evolve, allowing us to explore new horizons in computing and its applications.
Shor's Algorithm
Shor's Algorithm is a groundbreaking quantum algorithm for integer factorization. Developed by mathematician Peter Shor in 1994, it revolutionized the field by demonstrating that quantum computers can break widely used cryptographic protocols. The algorithm efficiently finds the prime factors of a composite number in a time complexity that is much lower than classical algorithms. This property poses a potential threat to current encryption methods, making it essential for cybersecurity.
The significance of Shor's Algorithm lies in two crucial implications:
- Cryptography Vulnerability: Classical encryption techniques, such as RSA, rely on the difficulty of factorizing large integers. Shor's Algorithm shows that quantum computers could easily break these encryptions, leading to consequences for data security.
- Advancement of Quantum Technology: Continued research into Shor's Algorithm may foster a deeper understanding of quantum mechanics and further enhancements in quantum processors,
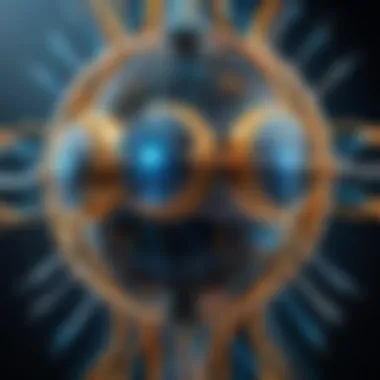
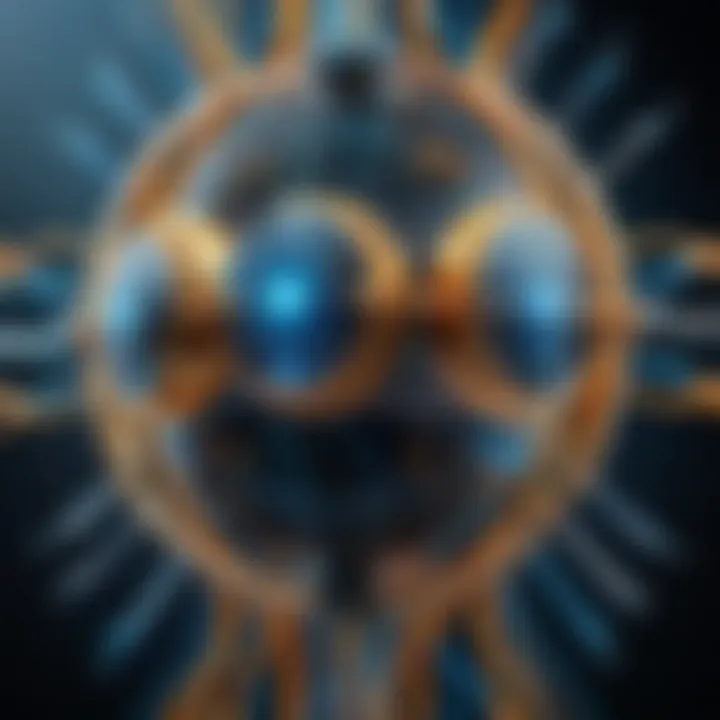
As quantum technologies progress, addressing the challenges posed by Shor's Algorithm will require re-evaluating the foundations of cryptography and exploring new methods for securing digital information.
Grover's Algorithm
Grover's Algorithm is another significant quantum algorithm, innovatively designed to search through an unsorted database or solve optimization problems with quadratic speedup as compared to classical algorithms. Introduced by Lov Grover in 1996, this algorithm allows for the unique benefit of finding a marked item among an unstructured database with an efficiency unattainable by classical search methods.
The core advantages of Grover's Algorithm include:
- Efficient Search: A search through ''N'' items is accomplished with only about √N evaluations, rather than N evaluations required classically.
- Broad Applicability: This algorithm is versatile, applicable in various areas including cryptography, database search, and AI.
However, Grover's Algorithm doesn't guarantee exponential speedup. It showcases the potential for optimization but also emphasizes the ongoing need for research to fully utilize this technique. Both Shor's and Grover's algorithms highlight the dual rise in risk and assistance that quantum computing brings to the processing of information.
Current State of Quantum Computing Technology
The realm of quantum computing technology is at a crucial juncture. Researchers and companies are focusing their efforts to develop practical quantum systems. This section delves into the significant players and recent breakthroughs in this exciting field, which holds immense potential for various applications.
Leading Players in the Field
Several key organizations and companies dominate the landscape of quantum computing. Their investements and innovations are shaping the future of this technology.
- IBM: Their Quantum Experience allows users to access their quantum processors via the cloud. They are pursuing superconducting qubits as their primary path to scalable quantum computers.
- Google: Their achievement of quantum supremacy in 2019 showcased the capability and promise of quantum entanglement. They focus on error correction and developing more powerful quantum processors.
- D-Wave Systems: They specialize in quantum annealing, highlighting the application of quantum computing to real-world problem-solving.
- Microsoft: They explore topological qubits to create more robust quantum systems, actively advancing the programming languages needed for these technologies.
- Rigetti Computing: Their forest platform connects quantum algorithms to classical computing systems, merging two important worlds seamlessly.
These players are competing to refine their quantum algorithms and create processors capable of outperforming classical supercomputers.
Breakthroughs and Milestones
Whenever a major breakthrough occurs in quantum computing, it often signifies a leap towards realization of practical applications. Currently, several prominent milestones demonstrate advances in the technology:
- Quantum Supremacy (2019): Google claimed this title, demonstrating a particular task that could be solved faster on a quantum computer than on traditional ones.
- IBM’s Quantum Volume (2020): IBM introduced an overall measure of its quantum system’s capability, which reflects the interconnectedness and accuracy of its quantum bits.
- Error Correction Initiatives: Ongoing efforts in quantum error correction are critical to enhancing stability in quantum computers. Successful implementations of such corretion techniques signify significant progress in making quantum systems practical.
Achieving practical quantum computing spans beyond just technical milestones. It also involves addressing the issues of stability, error rates, and usability.
Applications of Quantum Computing
Quantum computing is not just an academic curiosity; its applications carry the potential to revolutionize numerous industries. This section explores three critical applications: cryptography, drug discovery, and optimization problems. Understanding these applications offers insights into the transformative effects quantum computing may have on society and technology at large.
Cryptography
Cryptography stands as one of the most discussed applications of quantum computing. The world largely relies on traditional encryption methods to secure data. However, quantum computing introduces the possibility of sophisticated attacks on these systems.
One pivotal development is the concept of quantum key distribution (QKD), which utilizes the principles of quantum mechanics to create secure communication channels. Unlike classical methods where keys can be intercepted, QKD ensures that any eavesdropping is detectable, hence significant for maintaining security in digital communications.
The shift to quantum computers suggests that commonly used protocols, such as RSA and ECC, may become obsolete. This raises crucial discussions around
- Post-quantum cryptography: Developing algorithms resistant to quantum attacks.
- Transition strategies: Moving from classical systems to more secure quantum-enhanced security frameworks.
Drug Discovery and Material Science
In pharmaceuticals and material science, quantum computing shows immense promise in accelerating the discovery process. The molecular and chemical systems often thrive on vast computations that are computationally intensive, a situation traditional computers struggle with.
Quantum computers can perform simulations of molecular structures and reactions at a speed not feasible today. This capability leads to more pointed drugs in treatment therapies and innovative materials with unique properties. The considerations for this application include:
- Predicting molecular interactions with greater precision.
- Significantly reducing the time required for drug testing.
One of the exhilarating prospects is the development of personalized medicine, wherein treatments can be custom-tailored to genetic profiles based on efficient simulations. Companies like Rigetti Computing and IBM are conducting research on these applications in real-world settings.
Optimization Problems
Optimization problems are common in various fields, such as supply chain management, finance, and artificial intelligence. Industries continually focus on finding the most efficient solutions, often factoring complex constraints and variables. Classical algorithms function adequately; however, quantum computing could represent a stark improvement in some scenarios.
For instance, quantum annealers like those created by D-Wave Systems could help organizations resolve logistical questions more optimally than their classical counterparts. Specific uses illustrative of this are:
- Supply chain logistics: Organizing and optimizing delivery routes by analyzing numerous factors in real-time.
- Financial modeling and risk assessment: Managing and profiling risk in intricate investment structures.
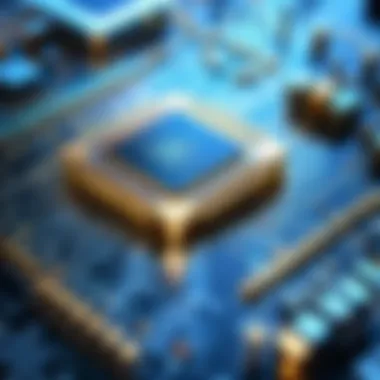
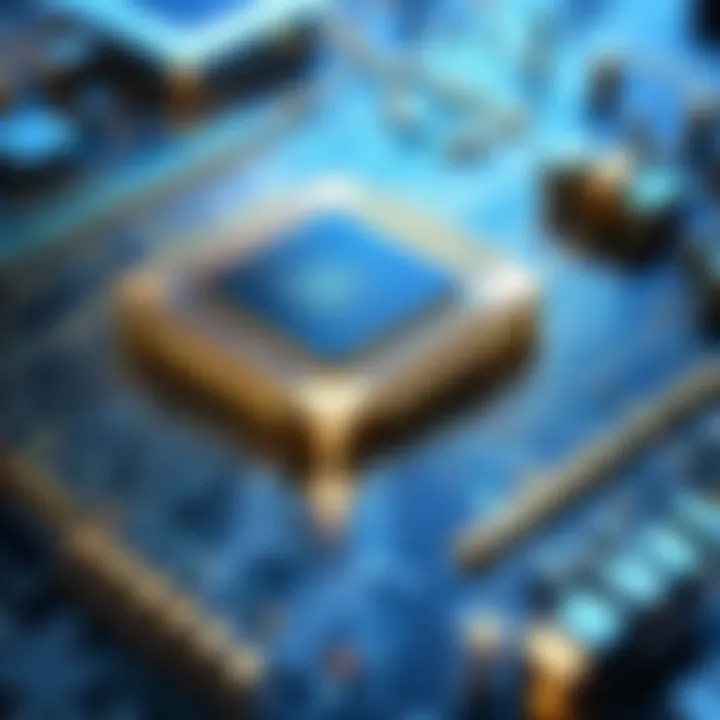
The implications for industry efficiency and cost savings are tremendous. Achieving significant advancements could also fast-track responses to ever-evolving market dynamics.
The exploration of quantum applications paints a landscape of opportunities that blend theoretical deep dive with practicality.
In summary, the applications of quantum computing stretch across numerous domains, marking critical advancements in cryptography, drug discovery, and optimization. Each area presents both opportunities and challenges that resonate throughout industry and technology today. Understanding these applications equips both enthusiasts and professionals with knowledge about the potential impact of quantum resarch.
Challenges in Quantum Computing
Understanding the challenges in quantum computing is essential for grasping the full scope of its potential. While quantum computing offers groundbreaking advancements, it is not without significant hurdles. These challenges can impact the development, scalability, and practicality of quantum systems. Addressing these issues requires innovative solutions and sustained research efforts.
Decoherence and Error Rates
Decoherence refers to the loss of quantum coherence, which is a fundamental property for executing quantum computations. When a qubit interacts with its surrounding environment, it tends to lose its superposition state, causing it to revert to classical conditions. This process creates errors in calculations.
Low error rates are crucial for building reliable quantum computers. Techniques such as error correction codes and fault-tolerant quantum computation are under active research. %E
Scalability Issues
Scalability is a major hurdle in practical quantum computing. Current quantum computers, while impressive, often have a limited number of qubits. Increasing the number of qubits while maintaining coherence and controlling error rates presents complications.
Quantum systems must manage larger qubit arrays without significantly increasing errors or decoherence. Furthermore, interconnections between qubits need precise control to avoid interference. To address scalability issues, researchers are working on modular systems and exploring different physical implementations.
Emerging designs may enhance connectivity and compatibility with classical systems while moving towards large-scale quantum computing. The path to scalable quantum systems remains conceptual at this point but crucial for practical applications.
Resource Requirements
Quantum computers demand distinctive resources compared to traditional computers. They require sophisticated infrastructure, including cryogenic systems to maintain low operational temperatures for certain qubit types, and substantial computational power for executing quantum algorithms.
Moreover, skilled personnel are needed to develop and maintain such intricate systems. The cost associated with developing quantum technologies is higher than conventional computing systems.
Efforts are being made to identify ways to reduce resource consumption and streamline operations. Innovative approaches like photonic quantum computing are promising as they might lead to less resource-intensive solutions.
The Future of Quantum Computing
The field of quantum computing stands on the brink of significant advancements, marking a new era for technology. Understanding the future prospects of this domain is crucial, as it intertwines not only with computational power but also with various aspects of everyday life. Quantum computing promises enhanced capabilities that traditional computing cannot achieve, allowing for complex problem-solving and optimizing various processes.
Emerging Trends and Research Areas
Researchers are currently focused on several key trends in quantum computing:
- Development of Quantum Hardware: Creating more stable qubits remains a high priority. Companies like IBM and Google invest significantly in innovations that can cut error rates associated with qubit manipulation.
- Quantum Software and Algorithms: Enhancing quantum algorithms is critical. New research is likely to yield algorithms capable of solving previously unsolvable problems. Prominent examples include quantum natural language processing or advancements in machine learning applications.
- Hybrid Quantum-Classical Models: There is a growing interest in employing hybrid models that leverage both classical and quantum computing, effectively merging the strengths of both systems for optimal performance.
Additionally, academic institutions invest into multidisciplinary research, exploring interactions between quantum tech and fields like cryptography, finance, and pharmaceuticals.
Potential Impact on Society
The societal implications of quantum computing are profound. Consider the following factors:
- Improvements in Cryptography: The ability of quantum computers to break traditional encryption exceeds classical systems. However, advanced cryptographic methods developed in response can offer heightened security.
- Healthcare Innovations: Quantum computing may revolutionize drug discovery. The ability to simulate molecular interactions precisely can catalyze the development of new medications.
- Addressing Climate Change: Quantum algorithms have the potential to aid in solving complex environmental models which can lead to technologies that mitigate climate change impacts.
The future of quantum computing hints at unprecedented growth and complexity. As society faces intricate challenges, leveraging quantum computing not only promises solutions but also reshapes how problems are understood and tackled. Its trajectory will determine not only future technological capabilities but also drive transformative changes across numerous industries.
The End
In any discussion around quantum computing, understanding its theoretical essence becomes vital. This article has traversed the complex constructions surrounding quantum computing, illustrating key insights and pondered potnential outcomes.
Recap of Key Insights
Major Concepts in Quantum Computing
- Quantum Bits (Qubits): The building blocks of quantum computing, permitting diverse states of computation unlike the classical bits.
- Superposition: This principle enables qubits to represent various states simultaneously, enhancing computational potential.
- Entanglement: A unique quantum trait facilitating instantaneous correlations, critical for the power of quantum computing.
Hazarding simplifications, relativity formulates that unlike classical computing, quantum computing possesses extenuated parallelism. These fatives potentially disrupt every industry characterized by computation-dependent operations, foreshadowing new paradigms.
Final Thoughts on Quantum Computing
The roadmap for quantum computing is not devoid of challenges. Beyond written complexities lays practical applicability conveyed through reliability barriers such as decoherence and resource demands for scalable infrastructures. Landmarks are being crafted continuously; however, strides emanate old concerns. Equipped with knowledge of its recalcitrant issues, quantum computing firms can better approach innovative solutions. With these considerations in mind, the field's optimistic predictions maintain persistence on achieving revolutionary technological advancements.
As researchers explore this territory, the future trajectory certainly appears laden with potential. Industry sectors stand poised for disruption, nudging forward possibilities once entertained solely as science fiction. Quantum computing, therefore, signifies more than just an advancement in machinery; it heralds the advent of transformative changes across scientific domains and societal functioning.